Research
Breadcrumb
- RIT/
- Biomechanical Imaging Lab/
- Research
General Methods for Elastic Inverse Problems
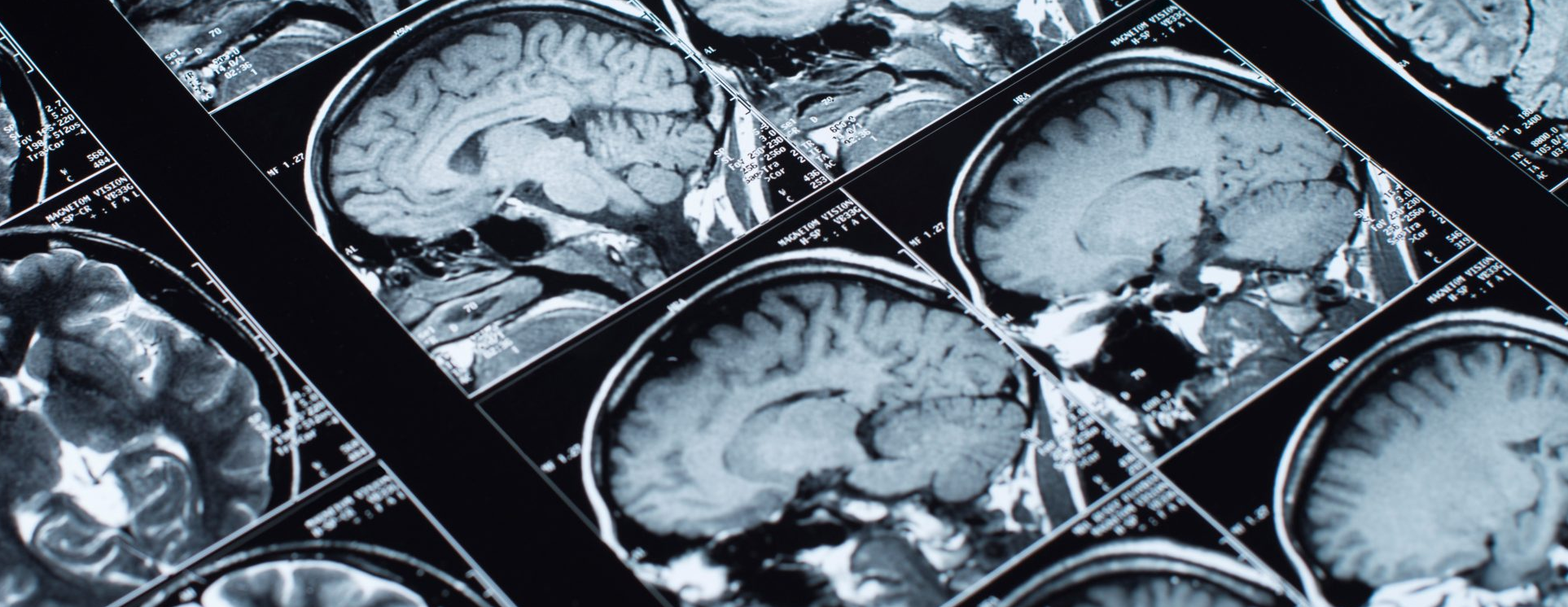
General Methods for Elastic Inverse Problems
Biomechanical Imaging (BMI) is a technique that expands upon the medical imaging practice of elastography to solve the inverse problem in solid mechanics. This technique takes displacement measurement as input and produces an estimate of a mechanical property field as an output. BMI provides a non-invasive means of characterizing tissue structure and function based on quantitative two or three-dimensional material property maps. Potential areas of application span both clinical (e.g. detection and diagnosis of cancerous tumors, atherosclerotic plaques, aneurysms, musculoskeletal disorders etc.) and laboratory settings (e.g. research tools for physiology and mechanobiology). Displacement measurements are commonly obtained by registration of images of tissue deformation acquired using medical imaging modalities such as ultrasound (US), magnetic resonance imaging (MRI), x-ray computed tomography, or optical coherence tomography. However, the inverse problem has many challenges that accompany it. For example, the appropriate choice of model (linear, non-linear, viscoelastic, etc.) depends on the type of deformation that is imposed on the tissue and subsequently measured. For the US, the most common method for data collection is a two-dimensional (2D) modality and, as such, 2D model assumptions, such as plane strain or plane stress, are required, even though it may be inaccurate. In addition, the elastic inverse problem is ill-posed, and the accuracy of the reconstruction can depend on the amount of input data, boundary condition assumptions and the choice of regularization. In general, our lab is investigating ways to improve the accuracy and utility of BMI by improving the models, methods and assumptions of BMI with a particular focus on clinical efficacy.
Risk Assessment of Abdominal Aortic Aneurysm
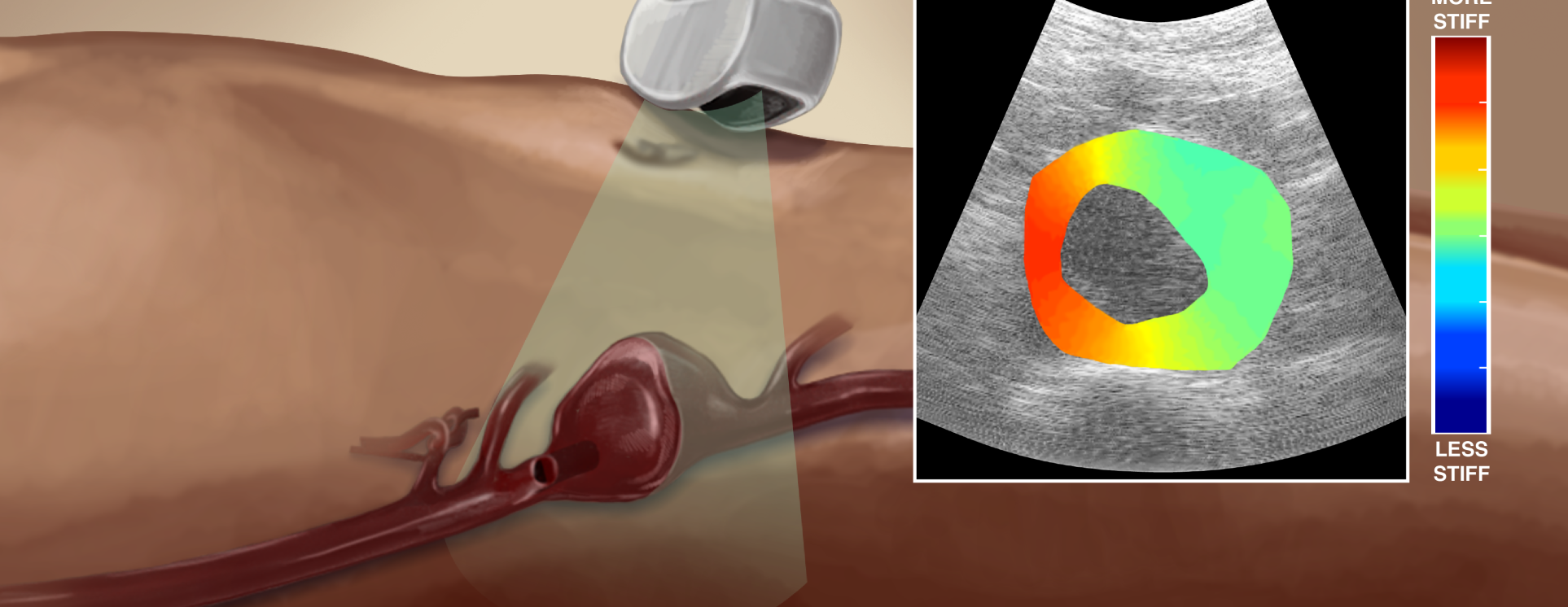
Risk Assessment of Abdominal Aortic Aneurysm
Aneurysms are an excessive localized enlargement of an artery caused by a weakening of the artery wall. For abdominal aortic aneurysms (AAA), clinicians rely on trans-abdominal ultrasound (US) measurements of the AAA maximum diameter. However, the AAA diameter is only a rough estimate of rupture potential. It has been suggested that measurement of stresses within the vessel wall may be crucial factor contributing to aneurysmal stability. Biomechanical imaging techniques can help us measure estimates of the material property changes within aortic tissue and potentially stresses within the wall. This technique is able to measure the aortic tissue stiffness in cross-sectional views of the vessel at the point of maximal diameter. Our lab uses a linear elastic, finite-element model to solve the elastic inverse problem and estimate the shear modulus in that cross-section. This technique also requires a non-invasive blood pressure measurement to estimate the pulse pressure in the aorta and normalize the modulus values. A model based, pulse wave velocity (PWV) technique is also being used to approximate the overall modulus value of the vessel wall in AAA. This technique uses ultrafast US imaging of the aorta in a longitudinal view to approximate the vessel properties.
In the video below (and linked here) we describe a method to manufacture aneurysmal, aortic tissue-mimicking phantoms for the use in testing ultrasound elastography. The combined use of computer-aided design (CAD) and 3-dimensional (3D) printing techniques produce aortic phantoms with predictable, complex geometries to validate the elastographic imaging algorithms with controlled experiments.
Ultrasound Elastography of Achilles Tendinopathy
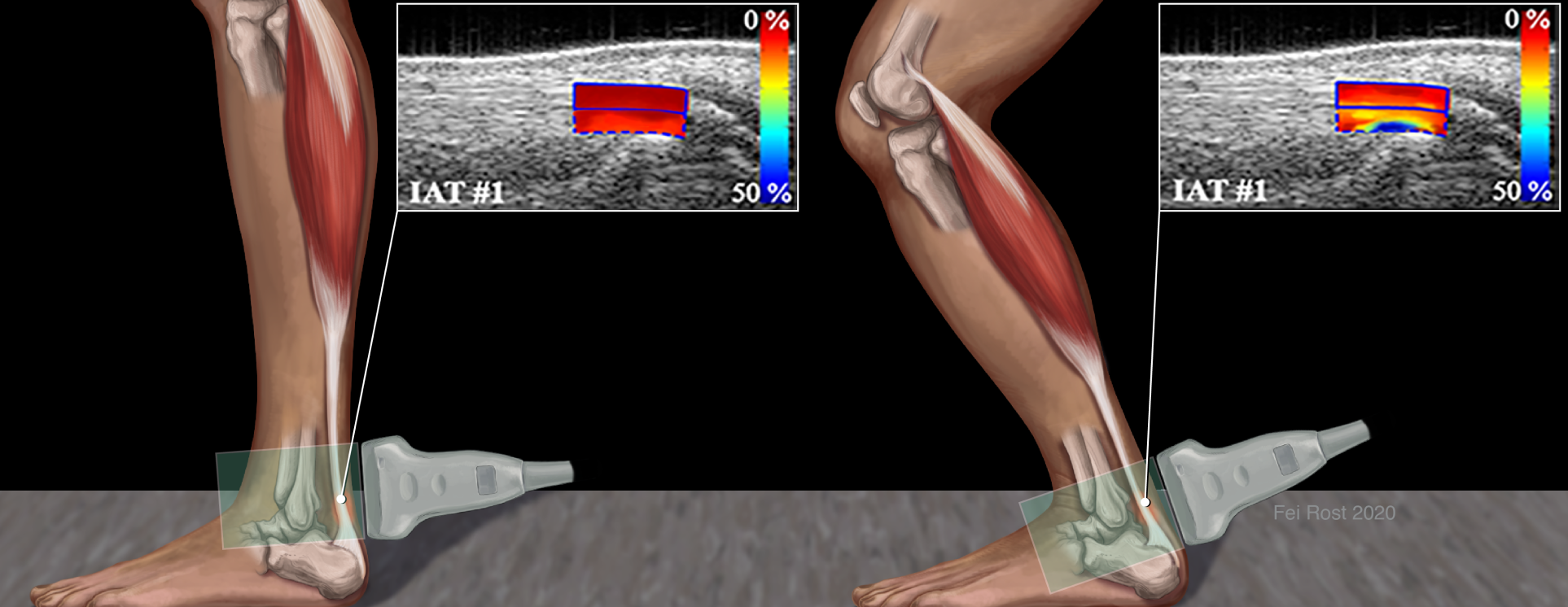
Ultrasound Elastography of Achilles Tendinopathy
Heel lifts are commonly prescribed to patients with Achilles tendinopathy with the general thought that it would relieve tendon stresses during walking to allow the tendon to heal. Yet little is known about the impact of tendon stress on the risk of injury or the healing process. The objective of this project is to demonstrate a connection between various biomechanical characteristics of tendons assessed in vivo via US imaging, including slack, buckling strain and stress in the Achilles tendon, to risk factors for tendinopathy. This work aims to develop an ultrasound elastography measurement protocol and algorithm to measure compressive strain of human Achilles tendon in vivo during certain exercises as well as the non-linear mechanical properties of the tendons for at risk populations. For example, it is well known that the risk of tendinopathy increases with age. It is our hypothesis that by understanding the mechanical properties and strains in the tendon as a function of aging, we may better understand the cause of this increased risk and potentially develop novel therapies based on our findings. Our techniques utilize common elastographic techniques such as quasi-static, quantitative elastography, measurement of large strains and shear wave imaging of tendons.
Development of a Longitudinal, Non-invasive Ultrasound Technique to Monitor Tendon Healing in a Mouse Model
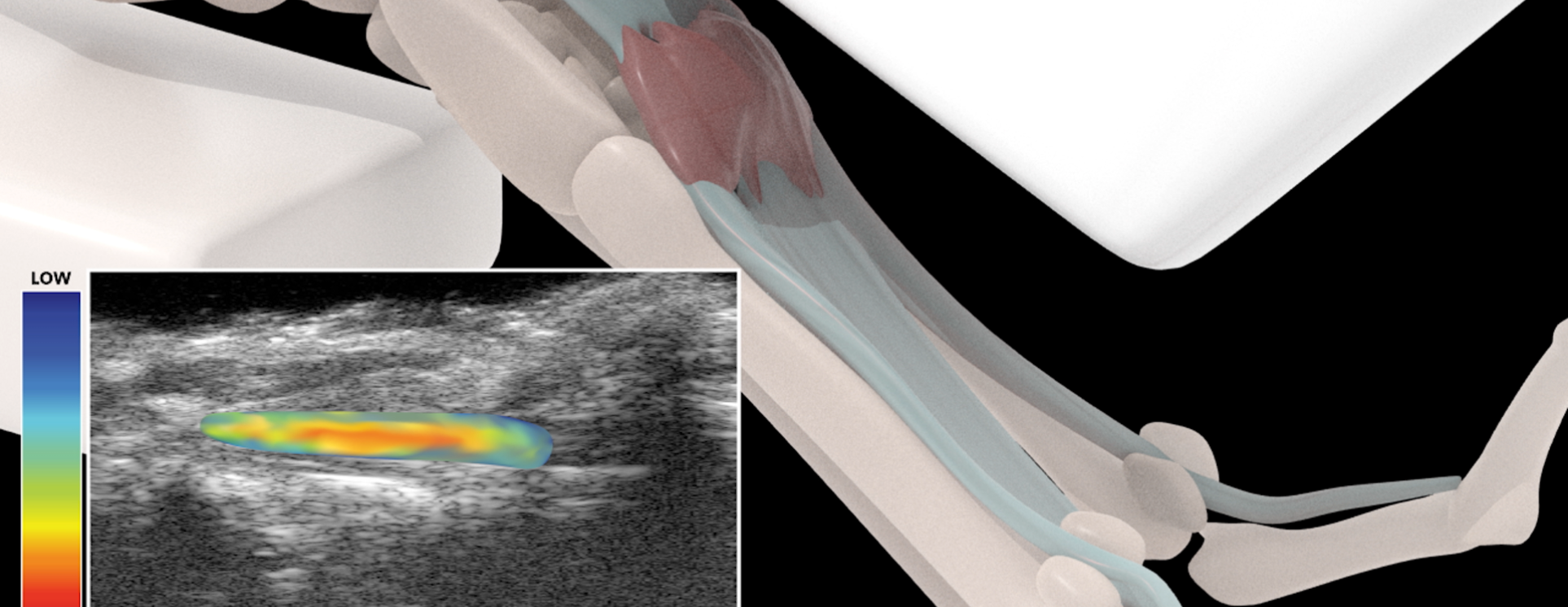
Development of a Longitudinal, Non-invasive Ultrasound Technique to Monitor Tendon Healing in a Mouse Model
Following surgical repair, tendon injuries heal with function-limiting and mechanically insufficient scar tissue. Despite attempts using a variety of biological and tissue engineering approaches, there is currently no consensus therapy to improve outcomes after tendon injury. An insufficient understanding of the underlying mechanisms that contribute to scar-mediated tendon healing is a major impediment to the development of successful therapies. To address this limitation, our collaborators use a murine model of tendon injury and repair that recapitulates many clinical aspects of healing including abundant scar tissue formation and impaired restoration of mechanical properties. However, there remains a need for cost-effective longitudinal outcome measures of tendon healing. For example, restoration of tendon gliding function, mechanical properties and tissue morphology are assessed by end-point analyses, which requires sacrificing the mice, and destructive testing of the tissue. Thus, current approaches are expensive, time-consuming and do not allow longitudinal evaluation or concomitant assessment of function and tissue morphology in the same specimen. This project aims to develop longitudinal, non-invasive metrics of tendon healing by combining ultrasound (US) elasticity imaging and novel image registration methods. US is an ideal modality to longitudinally assess tendon healing, as it is non-ionizing, and can be easily scaled between pre-clinical and clinical applications. Specifically, we are developing quantitative US outcomes of scar tissue volume, tendon excursion and tendon strain. Our hypothesis is that these metrics strongly correlate with terminal end-point parameters, and can therefore serve as faithful biomarkers of tendon healing. Once validated, this technology will permit the rapid screening of biological and pharmacological interventions for healing, and identify promising therapeutic targets in an efficient, cost-effective manner.