Research
Dr. Hubbard’s research focuses on Photovoltaic and Optoelectronic Devices, Radiation Hardened Space Power, III-V Semiconductors, and Vapor Phase Epitaxy.
Our activities encompass materials synthesis, device fabrication, material and device modeling, as well as characterization both at the electrical and materials level. Specific expertise lies in vapor phase epitaxy (VPE) of III-V photovoltaic devices and nanostructures, novel photovoltaic structure growth and design and all forms of photovoltaic characterization and simulation.
Interests
- Epitaxial Crystal Growth by Metalorganic Chemical Vapor Deposition (MOCVD)
- Semiconductor Device Design and Fabrication
- Thin-Film Characterization Techniques
- MOCVD System Design
- Nano-structures (quantum wires/dots) for enhanced efficiency photovoltaic cells.
- High Efficiency Nanostructured III-V Photovoltaics for Solar Concentrator Application
- Growth of semiconductor nanostructures using MOVPE growth techniques
- Novel Approaches to Power Conversion (alphavoltaics, thin-film III-Vs)
- Nanostructured gas/chemical sensors
Current Projects
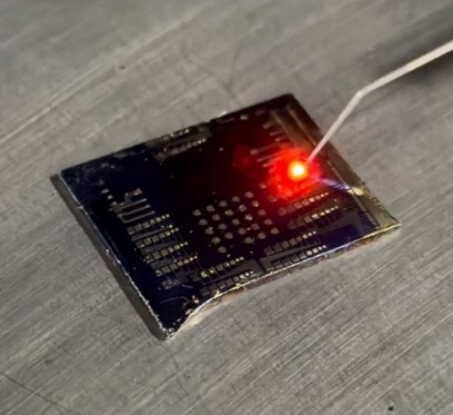
MOCVD regrowth of wide-bandgap (WBG) III-P Epilayers
The next generation of wearable devices will require small-featured displays to achieve high resolution. The micrometer light emitting diode (micro-LED) can enable near-eye displays through low power consumption, long lifetime, high contrast, enhanced response time and increased resolution. The micro-LED design proposes to significantly improve the resolution of near-eye displays since they are over 10-times smaller than conventional mm-size LED. However, the process development required to create a micro-LED damages the sidewalls and introduces defects, which degrades the light output power of these devices. As these devices reduce in size, surface states limit the light output power (LOP) at the roughened sidewalls, and the perimeter-to-area ratio must be considered. Light management through sidewall passivation, surface cleaning, and regrowth techniques are being studied to improve the LOP from these devices.
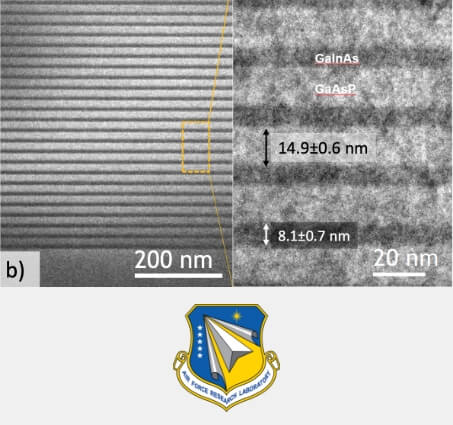
High Efficiency Quantum Structured Solar Cells
Nanostructured quantum well and quantum dot III–V solar cells provide a pathway to implement advanced single or dual junction photovoltaic device designs that can capture energy typically lost in traditional solar cells. A strained quantum well superlattice solar cell recently fabricated and tested at RIT achieved an AM1.5 efficiency greater than 30% with great promise for further improvements. A major opportunity exists to advance solar power generation and resiliency by exploring QW devices, increasing their TRL, and transferring technology to industry. QW structures can be implemented into many types of solar cell devices, potentially increasing power by up to 25% for satellites at end-of-life and 10% for aircraft, compared to state-of-practice (SOP) 3-junction devices.
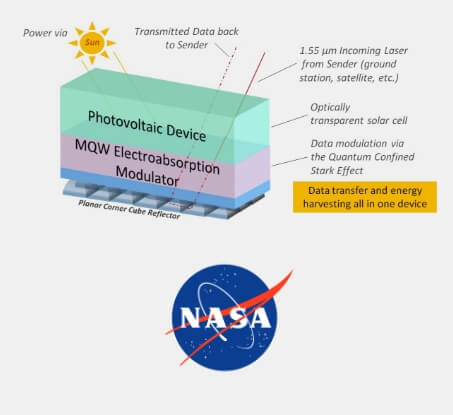
Solar Cell Integrated Modulating Retroreflector
One of the major drivers in recent and future satellite research is the need for more satellites and increased communication speed, especially with the emergence of proliferated low-Earth orbit (pLEO) constellations. The aerospace industry is now trending towards using optical data communication, as opposed to legacy radio frequency (RF) communications. III-V based multiple quantum-well (MQW) based electroabsorptive modulators (EAMs) have been used to demonstrate high speed, high ON/OFF keyed FSO communication. III-V semiconductors are also the class of materials typically used for photovoltaic (PV) arrays for satellites due to their exceptional efficiencies of upwards of 35% and ability to be made thin, flexible, and lightweight. PV has the potential to be an enabling platform for integrating an optical communication component for free space optical (FSO) systems, such as an EAM, due to the large aperture provided by the solar panels for a satellite and the already existing solar cell architecture. Combining an EAM with the large area PV array reduces the pointing accuracy required for FSO. Additionally, this reduces the SWaP of the system by hybridizing power and communication into one package. Our team is working on heterogeneous integration of FSO systems with PV using both mechanical and moonlight approaches.
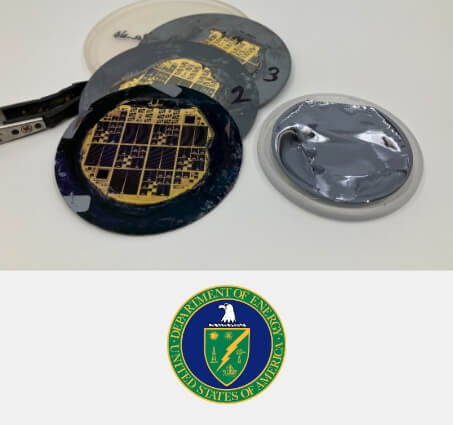
Sonic Wafering of III-V substrates for High Efficiency Cells: A path to <$0.50/W
III-V solar cells have long-demonstrated the highest power conversion efficiencies of any material system. However, a recent techno-economic analysis placed the fraction of GaAs single-junction photovoltaic device cost due to the substrate as high as 84%. Partners at Arizona State University and the National Renewable Energy Laboratory have been developing a wafer spalling technique that could have a direct impact on this high cost by cyclically removing the product device from the substrate, and enabling substrate re-use for additional PV manufacturing. The technique combines the prestressing of a GaAs crystal at low temperature with acoustic waves to controllably propagate a crack at low velocities. RIT is collaborating with our partners in the investigation of metalorganic chemical vapor deposition of GaAs on spalled substrates and fabrication of low cost, large area III-V solar cells.
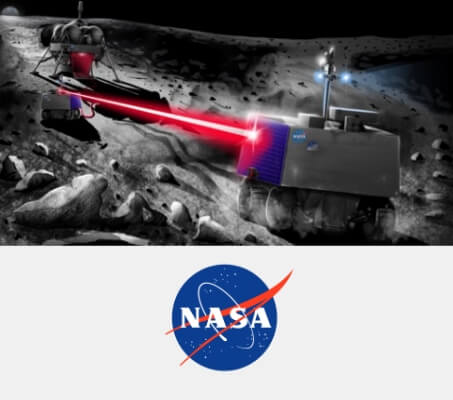
High Efficiency Laser Power Beaming Receiver for Lunar and Extraterrestrial Exploration
One of NASA’s strategic goals is a return to cis-lunar space and establishment of permeant manned and unmanned scientific installations on the lunar surface. Study of so-called “cold traps”, or permanently shadowed lunar craters near the north and south polar regions is of high importance since they may contain water ice, useful for both scientific study of the early history of the moon-Earth system as well as a potential source of hydrogen and oxygen for use in habitation or as fuel. However, the temperature of the lunar surface in the dark averages near 140K, and may be closer to 25K inside permanently shadowed craters. Currently, operational energy demands of these unmanned/autonomous lunar systems would be dependent on on-board energy storage of fuel or batteries which impose a time limit on operation. However, through appropriate wireless laser power beaming, the power reserves of a system could be extended indefinitely. Long-range power transfer in the far-field regime (meters to kilometer range) requires directional and concentrated sources of radiation available using lasers. Laser power beaming requires smaller size transmitters and receivers compared to microwaves; the systems can be built using all solid-state components; they have higher immunity to electromagnetic interference; and they have the potential for conversion efficiency over 70%. RIT is working on a number of goals to both grow multijunction power converters for 1064 nm operation as well as combining our converters with data transmission/reception devices to further impact SWaP.
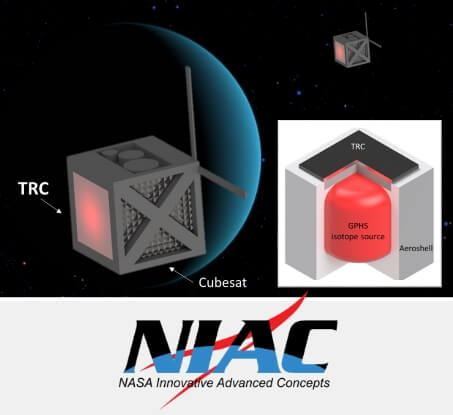
Radioisotope Thermoradiative Cell Power Generator
This NASA Innovative Advanced Concepts (NIAC) project investigates a revolutionary power source for missions to the outer planets utilizing a new paradigm in thermal power conversion, the thermoradiative cell (TRC). Available solar energy decreases as the square of the distance from the Sun, resulting in very low irradiance at the outer planets making photovoltaics infeasible. Instead, current missions beyond Jupiter are powered by radioisotope thermoelectric generators (RTG) which can only convert about 6% of the thermal energy produced by their expensive, and difficult to produce, plutonium heat sources. The TRC is a new alternative in solid state thermal to electrical energy conversion which operates like a solar cell in reverse. Photons are spontaneously generated by recombination of charge carriers inside the TRC diode which are rejected into cold outer space, thus cooling the TRC. A radioisotope heat source maintains the TRC temperature, allowing this radiative emission to drive a current inside the device and provide power to an external load. Low bandgap semiconductor materials are necessary for operation, leading RIT to investigate InAsSb at 0.28 eV. Our work suggests power conversion efficiencies in excess of 12% are possible in this system, doubling that of current state-of-the-art RTGs. Higher power conversion efficiency will enable better utilization of plutonium, and the reduced form factor of the TRC versus the RTG will allow use in many small cubesats as opposed to one large craft, increasing mission capabilities.
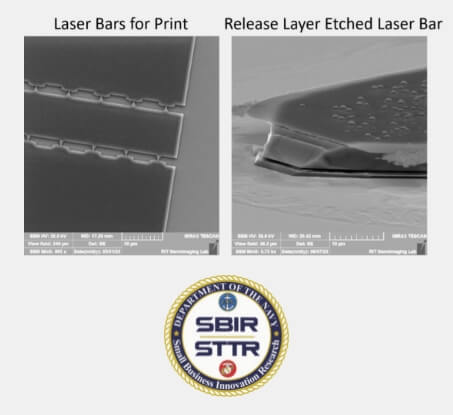
Hybrid and Heterogeneous Integration of PICs for RF Photonic Imaging Systems
The goal of this collaborative effort is to develop high-density heterogeneous and hybrid photonic integrated circuit (PIC) packaging techniques to support the demonstration of an analog RF photonic imaging system-in-a-package. Our team is primarily contributing to developing hybrid and heterogeneous integration of PIC materials to realize a multi-chip electro-optic system on-chip using Micro Transfer Printing (µTP) and photonic wire bonding (PWB).