Professors take new approach to science education
New approach to science education teaches critical thinking
A. Sue Weisler
This model represents the backbone of a protein. Colorful objects stuck to it represent amino acid side chains.
The Amino Acid Starter Kit looks like a toy. At first glance, the clear plastic pieces and colorful foam-covered wires don’t look like they belong in a college classroom.
Don’t be fooled. The three-dimensional models convey the complexity of amino acids and protein folding in ways that elude textbook illustrations. Assistant professor Dina Newman asked the students in her cell biology class to construct a coherent picture of a protein molecule for themselves. In order to build the molecule, they had to grapple with their understanding of its structure and follow the laws of chemistry.
The availability of commercial models such as the ones Newman uses in her class hint at new research-based approaches to teaching biology, a trend echoing throughout the College of Science at RIT and in institutions across the country. Scientists from within their own disciplines are discussing and conducting research about science education, once primarily the purview of schools of education.
Educating students in science, technology, engineering and mathematics, or the STEM disciplines, is a national concern. College of Science Dean Sophia Maggelakis cites the February 2012 report from the U.S. President’s Council of Advisors on Science and Technology, which calls for more graduates with STEM degrees.
“Cultivating the younger generation’s interest in science and mathematics is essential for ensuring that the United States remains a player in the research and development of new technology,” Maggelakis says. “We are falling behind and the importance of high quality STEM programs is widely recognized as both fundamental to educational initiatives and important to the country’s competitiveness and long-term economic health.”
Under Maggelakis’ leadership, the College of Science is moving to consolidate and grow its various STEM initiatives under a central nexus.
Nurturing young scientists through experiential learning—or “learning science by doing science”—is a priority for Anne Houtman, who joined RIT in 2011 as head of the recently named Thomas H. Gosnell School of Life Sciences. She oversees programs in biology, biotechnology, bioinformatics and environmental sciences. Nearly 500 students are enrolled in the programs, which draw approximately 100 new students each year.
“All of us who are involved with science education reform share a go-to idea,” Houtman says. “The goal you start with is to teach students to think like a scientist. And if you’re teaching non-scientists, the goal is to help students understand how scientists think and act so they have a better understanding for the process of science.”
Since 1994, Houtman has been an active member of Project Kaleidoscope, a national organization that focuses on science-education reform. Project Kaleidoscope advocates for strengthening undergraduate STEM education through experiential learning and critical assessment.
“We’re doing such an amazing job in the upper-level classes but our students really need to experience hands-on research opportunities from day one,” Houtman says.
Nick Fisk, a third-year student majoring in biology and biotechnology, appreciates the new approach.
“The way I see it, improving the methods and techniques used to instruct any life science course is vital to future student understanding of biology.”
Active learning
Redesigned introductory biology courses rolled out this past fall with built-in pre- and post-assessment surveys to gauge learning gains. A special section was developed for incoming majors who earned advanced placement credit in biology while in high school. Instead of repeating the class, this group of 24 students applies introductory concepts to a hot topic in the field—“vanishing amphibians.” The idea to focus on this topic came from Harvey Pough, professor of biology, who joined RIT in 2004 for the previous transformation of introductory biology.
“Advanced placement high school biology courses often use condensed versions of college textbooks, and students don’t like to be told that they must repeat that material,” Pough says. “In the AP Scholars course we tell them, ‘OK, you learned that material in high school, now you’re going to use it to do research.’ Vanishing amphibians is an ideal theme because it involves biological processes that extend from molecules to ecosystems.”
Newman and Kate Wright ’97 (biotechnology), assistant professor of biology, credit Pough’s approach of “learning biology by doing biology” as influential to their career development.
Next fall, Pough and Newman will be among the faculty teaching vanishing amphibians. Wright will be one of the professors leading the revised, yet more traditional, introductory course for the majority of incoming biology majors.
“Our philosophy is that it’s the same course content, it’s just delivered differently,” Wright says. “Many elements are the same: the investigative projects, experimental design, generating and communicating data, the discussion and the active learning.”
Houtman’s arrival at RIT coincided with a growing interest among a small group of faculty in science-education research and reform.
With the support of Dean Maggelakis and RIT Provost Jeremy Haefner, Scott Franklin, professor of physics, established the Science and Mathematics Education Research Collaborative to conduct discipline-based education research, curriculum development and advocate for evidence-based teaching practice as a metric of success. Members of the collaborative include Newman and Wright, Tom Kim, associate professor of biochemistry, and Robert Teese, professor of physics. Together, Houtman and the research group were influential in establishing the Project Kaleidoscope Upstate New York Regional Network, a branch of the parent organization.
Science-education reform—the trend toward discipline-based education research, experiential learning in the classroom and critical assessment of learning gains championed by Project Kaleidoscope—first took root in physics 40 years ago, Houtman says.
“Biology has been late to the table because we have so much content and it’s hard for faculty to let go of content and spend a little less time on the details of what’s inside a cell and a little more time to give students the chance to engage actively with their material,” she says.
Franklin, who earned his doctorate in physics, conducted physics education research from 1997–2000 during a National Science Foundation Postdoctoral Fellowship in Science, Mathematics, Engineering and Technology Education.
“Physics was the first of the disciplines to start taking a rigorous look at teaching and learning in ways that brought together education research, cognitive science and their discipline,” he says. “Chemistry is now doing it, biology is now doing it and math is beginning to. For whatever reason, physics is the one that started it in the early 1970s.”
Big ideas
A decade ago, RIT’s Department of Physics, now part of the School of Physics and Astronomy, changed how introductory physics is taught by switching up the classroom. Team Physics, which includes eight sections of university and college physics, threw out the traditional lecture format in favor of a workshop-like setting. Now, students collaborate in small groups on worksheets focusing on comprehension and problem solving.
“The workshop room allows students to have a lecture for 10 minutes and a lab for 10 minutes and then have a worksheet for 10 minutes and solve a problem,” Franklin says. “It’s much more flexible in terms of what you can do in a classroom.”
Franklin is a strong proponent of scientists conducting education research within their respective fields. “Discipline-based education research is all about combining social science theories and methods with a deep content knowledge to produce a new form of research,” he says. “You need to know the discipline to ask the right questions and to understand what you are observing in addition to using the curriculum in the right way and assessing if it’s being taught better.”
He considers the collaborative “an opportunity” for RIT to become a leader in discipline-based education research. The number of similar multidisciplinary research groups is small but growing. Pockets of science-education researchers are active at the Milwaukee School of Engineering, University of Maine, University of Colorado, Florida International, University of Illinois, University of Maryland and Rutgers University.
Members of the RIT collaborative are working to add the university’s name to that list through their awards, publications and national presentations.
Their work has already gained notice. In December, Franklin led an American Association for the Advancement of Science working group on research-based documentation of teaching practice.
Prior to that, in August, Franklin represented the Science and Mathematics Education Research Collaborative on a panel focused on interdisciplinary discipline-based education research groups at the national meeting of the American Association of Physics Teachers.
Franklin’s ideas about education research and reform resonate with Newman and Wright in biology, who share a deep interest in student learning and investigating classroom dynamics.
Their lectures also emphasize core concepts and overarching themes. Newman, a 2012 BEN Scholar, is developing resources for the BioSciEdNet, a digital library of biology educational materials managed by the American Association for the Advancement of Science.
“I try to get the students to see how there are patterns and how there are big ideas that come up over and over again,” says Newman. “For example, the structure-function relationship in proteins—or any biological molecule. The specific molecular structure is key to how it works. If you change the structure, you change the function. Some students do very well with that; other students are still trying to memorize the small details and missing the big picture.”
Two years ago, the National Science Foundation published a document called “Vision and Change: A Call to Reform Undergraduate Biology Education,” which outlined six core competencies that all undergraduate biology majors should know. The list includes the structure/function relationship that Newman reinforced in her cell biology class through the protein modeling activity.
“You focus on these concepts instead of trying to go over a ridiculous number of chapters in the textbook,” Wright says. “Because you can’t. I’ve also put the responsibility back on the students. They’re doing reading and online quizzes. In class, I like to focus on the key concepts—the main ideas. I’ve gone back and looked at my slides for my classes and I thought ‘Did I really go over 48 slides in the past? Why did I do that?’ I’m trying to do things differently.”
New approach
Back in Newman’s cell biology class, teaching assistant Christina Catavero stops at each table and talks to the students sticking amino acids to foam tubes representing protein backbones.
“I’m learning how to ask them what they know and not tell them the information,” says the third-year honors student from Poughquag, N.Y.
Catavero is majoring in biotechnology in the College of Science and biomedical sciences in the College of Health Sciences and Technology.
She published her first paper with Newman and Wright in the winter issue of CBE-Life Sciences Education about student failure to transfer their knowledge of chromosome structure to concepts of cell division. Catavero understands the value of using models like the Amino Acid Starter Kits to think about biological concepts on a different level.
“When students are hands on and doing it themselves, they’re making their own knowledge.”
Newman adds that it is an example of active learning.
“Research shows that students learn more when they take responsibility for their own learning,” she says. “It allows them to build new knowledge on the foundations of previous knowledge with input from peers.”
She looks around the room. “Notice no one has their phone out. They’re working together, talking and bouncing ideas off each other. It’s a much richer learning experience and it will stick with them longer than if I stood in front of the classroom and showed them how to construct a protein.”
The plastic-and-foam protein models have replaced the simple pipe cleaners and beads Newman and Wright used to hand out to their students.
Irene Evans, professor of biology, purchased several different models for the Thomas H. Gosnell School of Life Sciences with funding from a MERCK grant.
Other models include a water molecule kit, “the molecules of life”—plaster models of DNA, proteins, lipids and carbohydrates—a DNA starter kit, and gene maps—12-foot rolls in 12-point font.
“Models like these are fairly new,” Newman says. “But if you go back to Watson and Crick, they made their own model of DNA and, if you think about it, that’s the way to learn. Traditionally, this topic wouldn’t be taught this way. You get a very different picture when looking at a two-dimensional or three-dimensional drawing. Playing with the things is a way of learning. Questions you didn’t know to ask, you find the answers to.”
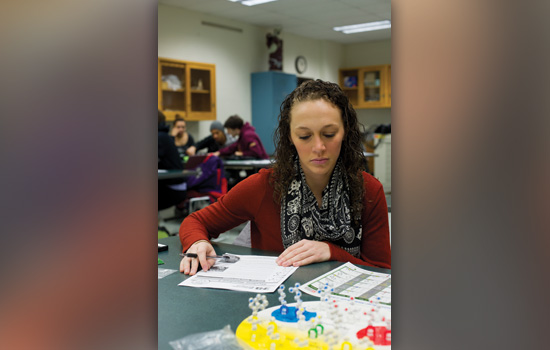
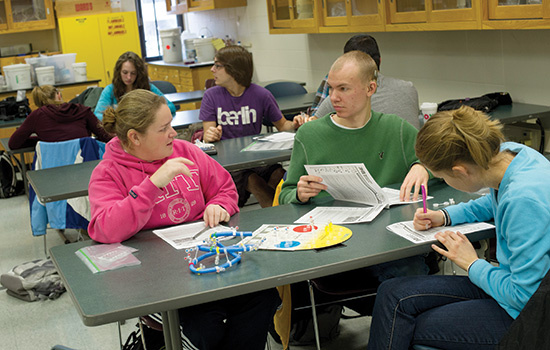
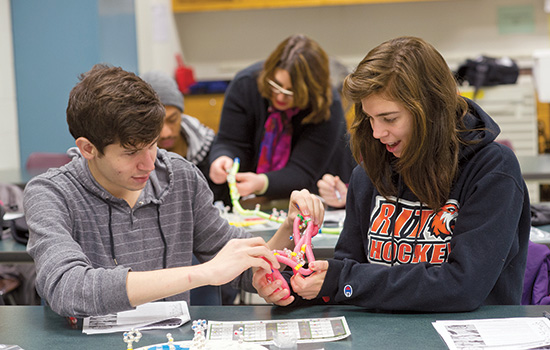
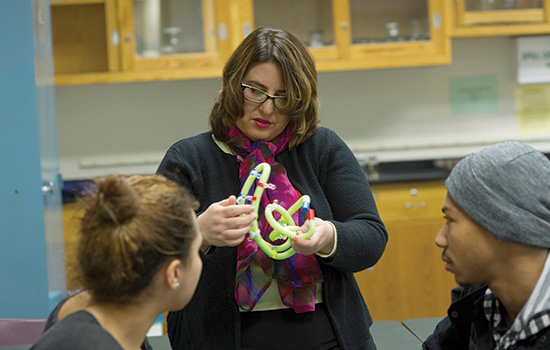